by neufer » Tue Jul 08, 2008 6:53 pm
http://chandra.harvard.edu/chronicle/04 ... index.html
<<The brightest supernova observed was the supernova of 1006 AD, which was brighter than Venus and visible for several years. However, the remnant of SN 1006 has not attracted nearly as much attention as the supernova of 1054 AD, which produced the Crab Nebula. This is because SN 1006 was likely produced by a thermonuclear explosion that completely disintegrated a white dwarf star (a Type Ia explosion), leaving nothing behind except the expanding stellar debris. In contrast, the Crab Nebula was the product of the collapse of the core of a massive star. The collapse triggered a Type II supernova that ejected most of the star, but left behind a rapidly spinning neutron star. This cosmic dynamo produces a tornado of magnetic fields and high-energy particles that continue to light up the expanding debris.>>
---------------------------------------------------
SN 1006 : 10 times brighter than Venus - visible for 3 years
SN 1054 (Crab) : bright as Venus - visible for 21 months
SN 1572 (Tycho) : bright as Venus - visible for 18 months
SN 1604 (Kepler) : brighter than Jupiter - visible for 12 months
---------------------------------------------------
http://en.wikipedia.org/wiki/Supernova
http://en.wikipedia.org/wiki/Type_Ia_supernova
Type Ia supernova [e.g., SN 1006]
<<There are several means by which a supernova of this type can form, but they share a common underlying mechanism. If a carbon-oxygen white dwarf accreted enough matter to reach the Chandrasekhar limit of about 1.38 solar masses (for a non-rotating star), it would no longer be able to support the bulk of its plasma through electron degeneracy pressure and would begin to collapse. However, the current view is that this limit is not normally attained; increasing temperature and density inside the core ignite carbon fusion as the star approaches the limit (to within about 1%), before collapse is initiated. Within a few seconds, a substantial fraction of the matter in the white dwarf undergoes nuclear fusion, releasing enough energy (1–2 × 1044 joules) to unbind the star in a supernova explosion. An outwardly expanding shock wave is generated, with matter reaching velocities on the order of 5,000–20,000 km/s, or roughly 3% of the speed of light. There is also a significant increase in luminosity, reaching an absolute magnitude of -19.3 (or 5 billion times brighter than the Sun), with little variation.
One model for the formation of this category of supernova is a close binary star system. The larger of the two stars is the first to evolve off the main sequence, and it expands to form a red giant. The two stars now share a common envelope, causing their mutual orbit to shrink. The giant star then sheds most of its envelope, losing mass until it can no longer continue nuclear fusion. At this point it becomes a white dwarf star, composed primarily of carbon and oxygen. Eventually the secondary star also evolves off the main sequence to form a red giant. Matter from the giant is accreted by the white dwarf, causing the latter to increase in mass.
Another model for the formation of a Type Ia explosion involves the merger of two white dwarf stars, with the combined mass momentarily exceeding the Chandrasekhar limit. A white dwarf could also accrete matter from other types of companions, including a main sequence star (if the orbit is sufficiently close).
Type Ia supernovae follow a characteristic light curve—the graph of luminosity as a function of time—after the explosion. This luminosity is generated by the radioactive decay of nickel-56 through cobalt-56 to iron-56. The peak luminosity of the light curve was believed to be consistent across Type Ia supernovae (the vast majority of which are initiated with a uniform mass via the accretion mechanism), allowing them to be used as a secondary standard candle to measure the distance to their host galaxies. However, recent discoveries reveal that there is some evolution in the average lightcurve width, and thus in the intrinsic luminosity of Supernovae, although significant evolution is found only over a large redshift baseline.>>
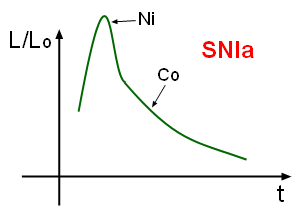
http://chandra.harvard.edu/chronicle/0406/historical_snr/index.html
<<The brightest supernova observed was the supernova of 1006 AD, which was brighter than Venus and visible for several years. However, the remnant of SN 1006 has not attracted nearly as much attention as the supernova of 1054 AD, which produced the Crab Nebula. This is because SN 1006 was likely produced by a thermonuclear explosion that completely disintegrated a white dwarf star (a Type Ia explosion), leaving nothing behind except the expanding stellar debris. In contrast, the Crab Nebula was the product of the collapse of the core of a massive star. The collapse triggered a Type II supernova that ejected most of the star, but left behind a rapidly spinning neutron star. This cosmic dynamo produces a tornado of magnetic fields and high-energy particles that continue to light up the expanding debris.>>
---------------------------------------------------
SN 1006 : 10 times brighter than Venus - visible for 3 years
SN 1054 (Crab) : bright as Venus - visible for 21 months
SN 1572 (Tycho) : bright as Venus - visible for 18 months
SN 1604 (Kepler) : brighter than Jupiter - visible for 12 months
---------------------------------------------------
http://en.wikipedia.org/wiki/Supernova
http://en.wikipedia.org/wiki/Type_Ia_supernova
Type Ia supernova [e.g., SN 1006]
<<There are several means by which a supernova of this type can form, but they share a common underlying mechanism. If a carbon-oxygen white dwarf accreted enough matter to reach the Chandrasekhar limit of about 1.38 solar masses (for a non-rotating star), it would no longer be able to support the bulk of its plasma through electron degeneracy pressure and would begin to collapse. However, the current view is that this limit is not normally attained; increasing temperature and density inside the core ignite carbon fusion as the star approaches the limit (to within about 1%), before collapse is initiated. Within a few seconds, a substantial fraction of the matter in the white dwarf undergoes nuclear fusion, releasing enough energy (1–2 × 1044 joules) to unbind the star in a supernova explosion. An outwardly expanding shock wave is generated, with matter reaching velocities on the order of 5,000–20,000 km/s, or roughly 3% of the speed of light. There is also a significant increase in luminosity, reaching an absolute magnitude of -19.3 (or 5 billion times brighter than the Sun), with little variation.
One model for the formation of this category of supernova is a close binary star system. The larger of the two stars is the first to evolve off the main sequence, and it expands to form a red giant. The two stars now share a common envelope, causing their mutual orbit to shrink. The giant star then sheds most of its envelope, losing mass until it can no longer continue nuclear fusion. At this point it becomes a white dwarf star, composed primarily of carbon and oxygen. Eventually the secondary star also evolves off the main sequence to form a red giant. Matter from the giant is accreted by the white dwarf, causing the latter to increase in mass.
Another model for the formation of a Type Ia explosion involves the merger of two white dwarf stars, with the combined mass momentarily exceeding the Chandrasekhar limit. A white dwarf could also accrete matter from other types of companions, including a main sequence star (if the orbit is sufficiently close).
Type Ia supernovae follow a characteristic light curve—the graph of luminosity as a function of time—after the explosion. This luminosity is generated by the radioactive decay of nickel-56 through cobalt-56 to iron-56. The peak luminosity of the light curve was believed to be consistent across Type Ia supernovae (the vast majority of which are initiated with a uniform mass via the accretion mechanism), allowing them to be used as a secondary standard candle to measure the distance to their host galaxies. However, recent discoveries reveal that there is some evolution in the average lightcurve width, and thus in the intrinsic luminosity of Supernovae, although significant evolution is found only over a large redshift baseline.>>
[img]http://upload.wikimedia.org/wikipedia/commons/8/88/SNIacurva.png[/img]